Energy transition at risk: The imperative to innovate in mining
by Dr. Isabella Fandrych, General Partner at Nucleus Capital.
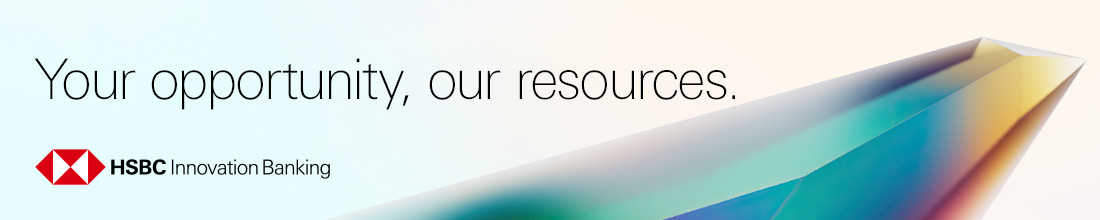
Guest post by Dr. Isabella Fandrych, General Partner at Nucleus Capital. | Originally published on Medium.
The need for resources
Decarbonizing our energy system necessitates a fundamental shift in its underlying material composition. In this new paradigm, energy is stored and transmitted through metals and minerals rather than hydrocarbons derived from fossil fuels. These minerals offer crucial attributes, enabling superior functionalities in energy capture, storage, and distribution. Because of these attributes, they are pivotal in enabling green technologies such as wind turbines, solar photovoltaic (PV) systems, batteries, and energy grids.
Therefore, the expansion of climate technologies is one of the most significant drivers of demand growth for these minerals. Due to their vital role in these components, their irreplaceability, and the strain on supply chains caused by geopolitical asymmetries in their distribution, these materials are referred to as ‘critical’ minerals.
For this article, the projected demand for critical minerals is grounded in three key scenarios provided by the International Energy Agency (IEA)². These scenarios are based on varying levels of effort in reducing greenhouse gas emissions to limit global warming in the coming decades, directly impacting the implementation of climate technologies and, thus, the demand for critical minerals.
Baseline Scenario (Stated Policies Scenario — STEPS): Only reflects current policies, i.e., assuming no additional policies beyond those already in place for reducing emissions and expanding clean technologies. This scenario is estimated to result in >2.5°C global warming by 2100.
Moderate Scenario (Announced Pledges Scenario — APS): Assumes all current climate commitments, including Nationally Determined Contributions (NDCs) and net-zero targets, are fully implemented on time. This scenario is estimated to result in >2°C of global warming by 2100.
Ambitious Scenario (Net Zero Emissions by 2050 Scenario — NZE): Outlines a pathway to achieve net-zero CO₂ emissions by 2050, with extensive deployment of clean technologies. This scenario is estimated to align with limiting global warming to 1.5°C by 2100.
Each scenario outlined necessitates a massive expansion of clean energy technologies, leading to a significant increase in demand for critical minerals such as lithium, graphite, nickel, cobalt, copper, and rare earth elements (REEs), as detailed in the following diagrams.
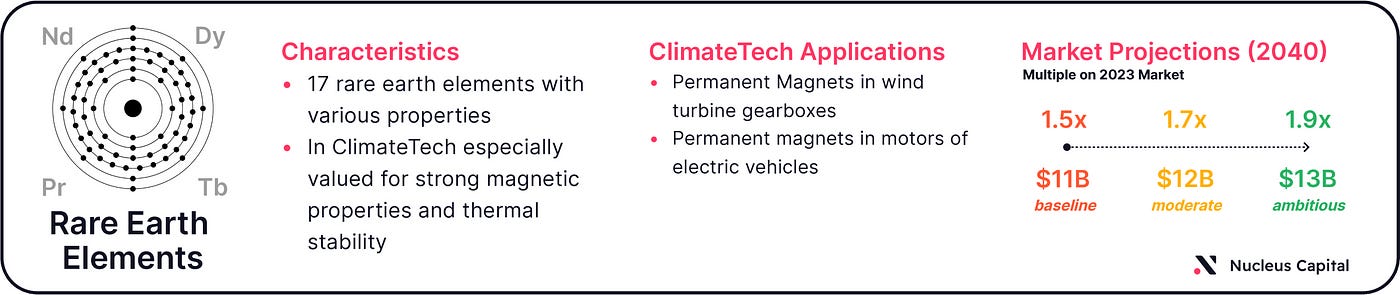
Extractability challenges let supplies grow scarce
At present, we can anticipate a surge in demand for minerals to enable the green transition, leading to a significant supply gap across a range of critical minerals in the anticipated scenarios.
Global mineral reserves are limited, and not all can be economically extracted using current methods. The world has largely tapped the easiest-to-extract deposits, leading to higher costs as mining companies must exert more effort to extract lower-quality minerals. Copper is a prime example of this trend: Today, copper ore typically contains only 1% or less copper, compared to 150 years ago when ore grades often exceeded 5%, with grades as high as 10% in rich mines¹⁶. This significant drop in availability of high-quality extractable ore necessitates extensive processing, driving up costs¹⁷.
“Commitment to the Paris Climate goals implies an intensifying drive — and competition — in the 21st century for the raw materials needed to achieve those goals. The ensuing scramble may be compared to that for fossil fuels in the 20th century. But copper production is more concentrated than oil.”
— The Future of Copper (S&P Global, 2022)¹⁶
Furthermore, 70% of global copper deposits are locked in low-grade chalcopyrite, a resource so impure that it is deemed too energy-intensive and expensive to mine with current methods, effectively making it inaccessible to the current world market¹⁶.
Currently planned mining efforts could leave the world in 2040 with a yearly lithium supply gap of 918,000 tons²², equivalent to approximately $152 billion²² in market value — accompanied by an enormous price increase
The need for regional independence
Moreover, the considerable geographic concentration along global value chains further exacerbates the anticipated price volatility. Currently, the supply of refined lithium is dominated by three countries, with Chinese companies alone supplying 65%≤²⁴ of global production.
China further provides 90%²⁴ of the current global graphite supply, including all battery-grade materials. In the moderate scenario, the IEA expects that by 2030, demand for graphite will be 10x¹¹ of the supply available outside of China, giving the country immense leverage over the conditions and opportunities for the rest of the world to achieve its energy transition goals⁸. Furthermore, high dependence on one geographic region increases the risk exposure to supply chain disruptions (e.g., think transportation and logistics during the Covid-19 pandemic).
The sustainability paradox
Simultaneously, sourcing critical minerals essential for the green transition presents significant sustainability challenges, impeding environmentally responsible scaling of mineral production. Mining operations contribute 5–14% of global greenhouse gas emissions²¹, primarily due to fossil fuel use in equipment and energy-intensive ore processing. This creates a paradoxical situation where the minerals essential for clean energy technologies have a substantial carbon footprint in their extraction and refinement.
Beyond emissions, mining activities have extensive environmental impacts. Large-scale mining leads to deforestation and habitat destruction, causing displacement or extinction of plant and animal species, some rare or endangered. Processing lower-grade ores demands increasingly energy-intensive methods, further expanding the sector’s environmental footprint. Mining also consumes vast quantities of water and can pollute water sources through acid mine drainage and release of heavy metals. Additionally, chemical use in extraction and processing releases toxic substances, endangering ecosystems and human health.
A new imperative for mineral sourcing
The future expansion of clean technologies in the face of climate change is uncertain. Given the substantial primary supply gap caused by non-cost-effective extraction methods that render most of the world’s reserves economically unviable, combined with the significant geopolitical risks already threatening the existing supply chains, it is imperative to evaluate alternative material sourcing methods. This also includes developing approaches to unlock currently inaccessible minerals found in complex material deposits, for example, in low concentrations among a wide range of other metals in impure brines. This would allow us to unlock new deposits not only across quality but also across geographies, effectively decentralizing sourcing capabilities. For instance, tapping into local lithium-rich clay sources in North America could significantly bolster regional supply chains.
In light of the steep increase in the supply and demand gap, businesses, policymakers, innovators, and investors need to find reliable ways to mitigate the anticipated supply crisis. Contributing to limiting the median global surface temperature increase to 1.5°C, the energy sector would increase global critical mineral demand until 2040 by 80%, elevating the global market to $770B.⁶ All actors have the unique opportunity to tap into an enormous market while driving immense impact on emission reduction targets and combating anthropogenic climate change. We expect more investors and corporates to pay stronger attention to mineral sourcing and already see an increase in early-stage companies started in this space.
If you’re a technologist working on innovative solutions along the critical mineral supply chain, please reach out to Isabella (isabella@nucleus-capital.com) or submit your deck to our website!
References
McKinsey & Company. 2022. “The Raw-materials Challenge: How the Metals and Mining Sector Will Be at the Core of Enabling the Energy Transition.” January 10, 2022. Accessed July 16, 2024. https://www.mckinsey.com/industries/metals-and-mining/our-insights/the-raw-materials-challenge-how-the-metals-and-mining-sector-will-be-at-the-core-of-enabling-the-energy-transition.
IEA (2021), World Energy Outlook 2021, IEA, Paris https://www.iea.org/reports/world-energy-outlook-2021. Accessed July 16, 2024.
IEA (2021), The Role of Critical Minerals in Clean Energy Transitions, IEA, Paris https://www.iea.org/reports/the-role-of-critical-minerals-in-clean-energy-transitions, Licence: CC BY 4.0. Accessed July 16, 2024.
IPCC. 2023. “Climate Change 2023: Synthesis Report.” Edited by Core Writing Team, H. Lee, and J. Romero. Accessed July 17, 2024. https://doi.org/10.59327/IPCC/AR6-9789291691647.
IEA (2021), The Role of Critical Minerals in Clean Energy Transitions, IEA, Paris https://www.iea.org/reports/the-role-of-critical-minerals-in-clean-energy-transitions, Licence: CC BY 4.0. Accessed July 17, 2024.
IEA (2024), Market value of key energy transition minerals in the Announced Pledges Scenario and the Net Zero Scenario, 2023–2040, IEA, Paris https://www.iea.org/data-and-statistics/charts/market-value-of-key-energy-transition-minerals-in-the-announced-pledges-scenario-and-the-net-zero-scenario-2023-2040, Licence: CC BY 4.0.
Methodology to calculate STEPS market size: Demand in 2040 in STEPS scenario x APS 2040 ton value (= 2040 APS market value / demand in tons).Goldman Sachs. 2023. “Direct Lithium Extraction: A Potential Game Changing Technology.” Report. Accessed July 17, 2024. https://www.goldmansachs.com/intelligence/pages/gs-research/direct-lithium-extraction/report.pdf.
McKinsey & Company . 2023. “Battery 2030: Resilient, Sustainable, and Circular.” January 16, 2023. Accessed July 17, 2024. https://www.mckinsey.com/industries/automotive-and-assembly/our-insights/battery-2030-resilient-sustainable-and-circular.
IEA (2024), Lithium, IEA, Paris https://www.iea.org/reports/lithium, Licence: CC BY 4.0. Accessed July 17, 2024.
The World Bank. 2020. “Minerals for Climate Action: The Mineral Intensity of the Clean Energy Transition.” The World Bank. International Bank for Reconstruction and Development/The World Bank. Accessed July 17, 2024. https://pubdocs.worldbank.org/en/961711588875536384/Minerals-for-Climate-Action-The-Mineral-Intensity-of-the-Clean-Energy-Transition.pdf.
IEA (2024), Graphite, IEA, Paris https://www.iea.org/reports/graphite, Licence: CC BY 4.0. Accessed July 17, 2024.
Nickel Institute. n.d. “About Nickel.” Accessed July 17, 2024. https://nickelinstitute.org/en/nickel-applications/#properties.
IEA (2024), Nickel, IEA, Paris https://www.iea.org/reports/nickel, Licence: CC BY 4.0. Accessed July 17, 2024.
Cobalt Institute. 2023. “Batteries & Electric Vehicles — Cobalt Institute.” November 5, 2023. Accessed July 17, 2024. https://www.cobaltinstitute.org/essential-cobalt-2/powering-the-green-economy/batteries-electric-vehicle/.
IEA (2024), Cobalt, IEA, Paris https://www.iea.org/reports/cobalt, Licence: CC BY 4.0. Accessed July 17, 2024.
S&P Global, Mohsen Bonakdarpour, and Tabitha M. Bailey. 2022. “The Future of Copper.” Accessed July 17, 2024. https://cdn.ihsmarkit.com/www/pdf/0722/The-Future-of-Copper_Full-Report_14July2022.pdf.
McKinsey & Company. 2023. “Bridging the Copper Supply Gap.” February 17, 2023. Accessed July 17, 2024. https://www.mckinsey.com/industries/metals-and-mining/our-insights/bridging-the-copper-supply-gap.
IEA (2024), Copper, IEA, Paris https://www.iea.org/reports/copper, Licence: CC BY 4.0. Accessed July 17, 2024.
McKinsey & Company. 2023. “Bridging the Copper Supply Gap.” February 17, 2023. Accessed July 17, 2024. https://www.mckinsey.com/industries/metals-and-mining/our-insights/bridging-the-copper-supply-gap.
Gielen, Dolf, and Martina Lyons. 2022. Critical Materials for the Energy Transition: Rare Earth Elements. International Renewable Energy Agency. https://www.irena.org/-/media/Irena/Files/Technical-papers/IRENA_Rare_Earth_Elements_2022.pdf?rev=6b1d592393f245f193b08eeed6512abc.
a.) McKinsey & Company. 2020. “Climate Risk and Decarbonization: What Every Mining CEO Needs to Know.” January 28, 2020. Accessed July 17, 2024. https://www.mckinsey.com/capabilities/sustainability/our-insights/climate-risk-and-decarbonization-what-every-mining-ceo-needs-to-know. | b.) IEA (2023), CO2 Emissions in 2022, IEA, Paris https://www.iea.org/reports/co2-emissions-in-2022, Licence: CC BY 4.0. Accessed July 17, 2024. | Methodology: McKinsey & Company estimates the CO₂-equivalent GHG emissions of the global mining sector to be between 1.9 and 5.1 gigatons. The IEA estimates global emissions in 2022 to 36.8 gigatons of CO₂-equivalents.
a.) IEA (2024), Critical Minerals Data Explorer, IEA, Paris https://www.iea.org/data-and-statistics/data-tools/critical-minerals-data-explorer. Accessed July 17, 2024. | b.) IEA (2024), Market value of key energy transition minerals in the Announced Pledges Scenario and the Net Zero Scenario, 2023–2040, IEA, Paris https://www.iea.org/data-and-statistics/charts/market-value-of-key-energy-transition-minerals-in-the-announced-pledges-scenario-and-the-net-zero-scenario-2023-2040, Licence: CC BY 4.0. | Methodology: The market size for lithium in 2040 in the APS scenario is estimated at $219B, equivalent to a demand of 1.326M tons. Based on current and announced projects, global supply capacities will be at 408k tons in 2040, leaving a 918k ton gap, proportional to a market value of $152B.
IEA (2023), Share of top three producing countries in mining of selected minerals, 2022, IEA, Paris https://www.iea.org/data-and-statistics/charts/share-of-top-three-producing-countries-in-mining-of-selected-minerals-2022, Licence: CC BY 4.0. Accessed July 17, 2024.
IEA (2023), Share of top three producing countries in processing of selected minerals, 2022, IEA, Paris https://www.iea.org/data-and-statistics/charts/share-of-top-three-producing-countries-in-processing-of-selected-minerals-2022, Licence: CC BY 4.0. Accessed July 17, 2024.
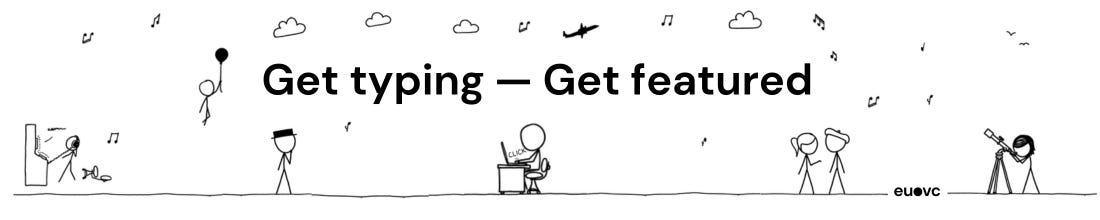